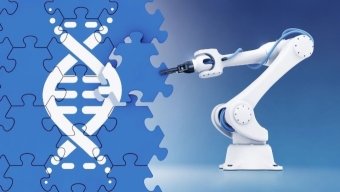
Researchers in UC Santa Barbara’s newly designated Bioengineering (BioE) Department have received a significant boost from the U.S. Army, which awarded the university a $9.85 million grant to design and purchase state-of-the-art equipment that project leader Michelle O’Malley, a professor of chemical engineering and biological engineering, says “allows UCSB scientists to do things that we never thought were possible.” The funding, awarded through the Department of Defense’s Defense University Research Instrumentation Program (DURIP), will allow the university to embrace a trend that has enabled pharmaceutical and biomedical companies to maximize their outputs and profits: automation.
“Synthetic biology is not limited by technology anymore,” said O’Malley, referring to the field of science that involves redesigning and engineering living systems and organisms, such as DNA and cells, to solve grand challenges in health and the environment. “It’s limited by a throughput bottleneck, because at the end of the day, a researcher still has only two hands and a finite number of hours to spend in a lab.”
The bottleneck exists because much of the work in a typical university research laboratory relies on manual manipulation of biology by undergraduate and graduate students, postdoctoral researchers, and technical staff as they manually carry out protocols and repetitive procedures, such as using pipettes.
“As a result, most researchers are never able to design, build and test enough prototypes to quickly move biotechnology forward,” said O’Malley, vice-chair of UCSB’s new Biological Engineering Department. “Automation eliminates the bottleneck and allow us to develop and analyze incredibly large datasets to find true breakthroughs.”
The university will purchase a workflow of robotic assembly and analytical tools to enable automated synthetic biology and establish the UCSB BioFoundry. The equipment was custom-designed for UCSB’s life science research community, shaped by discussions with more than forty principal investigators on campus. One system is designed to handle mammalian cells — the type of cells that researchers use as platforms for drug discovery or to learn how human cell biology works. A second set of robotic instrumentation will allow for rapid screening and engineering of microbial cells and microbial communities, such as bacteria, yeast, or fungi, which could be engineered to produce valuable products or clean up chemical contaminants in the environment. Both systems have broad biomedical and environmental applications. Each system will consist of several interwoven modules, which include: an integrated DNA foundry for genome design and editing, colony-picking and sorting capabilities, UV-Vis/fluorescence plate readers and plate incubators for strain growth, a high-speed mass spectrometry system, and high-speed imaging capabilities. The technology will operate without human intervention and allow for the miniaturized cultivation of cells with a menu of options to sample, test, and analyze. The equipment will be housed in two separate locations on campus to avoid cross-contamination between the two systems.
“These systems will allow us to pursue a wide range of research in areas where we have a significant amount of expertise on campus,” explained O’Malley. “We will have a robot used mostly to answer medical and cell biology questions and develop new biomedical technologies, and another one to accelerate knowledge and technology that will aid our goals related to environmental, sustainability, and biomanufacturing. That really encompasses the main pillars of strength in our Bioengineering Department, which is unique from other departments that focus solely on the medical space.”
The automated systems will generate massive amounts of data that can be used to train artificial intelligence (AI) algorithms. Researchers will be able to devote more time to analyzing the data and designing experiments without having to do the tedious work, which will be completed by the robotic workflows. They will be able to computationally design strains of cells for a certain outcome, and then, in a process similar to how evolution works, use the robotic workflow to prototype and evaluate thousands of possible configurations and growth conditions to identify the “best” strain. Researchers will also be to learn from engineered strains that are not successful.
“This gives us the kind of experimental firepower of a mid-size biotechnology or pharmaceutical company, but in a way that we can be flexible in pursuing answers to many different problems,” said Max Wilson, an assistant professor in the Molecular, Cellular, and Development Biology Department. “When you have robots that don’t sleep and don’t make mistakes, you can do extremely precise research at unprecedented scales.”
Wilson’s research group works to better understand how human cells make complex decisions so that they can engineer and control those cell behaviors for biomedical purposes. In particular, his group leverages synthetic biology tools to design novel light-responsive molecules that they use to program cells using pulses of light. They then use the information gained from these experiments to build advanced mathematical models that predict complex cell behaviors so that they can be more faithfully engineered.
“My lab specializes in using light to communicate with cellular systems. We will be able to use the new equipment to engineer photo-responsive proteins that we use to communicate with cells at an unprecedented scale,” explained Wilson, “We should get proteins that are much more efficient and be able to control extremely relevant processes that we are interested in engineering.”
His group will also be able to complete small-molecule drug screens and examine large sums of chemical matter to identify drugs that affect a disease or a phenotype.
Wilson and O’Malley believe that the new facilities will give students a unique experience of learning how to interact with automation, which is the next wave in biotechnology. That real-world experience, coupled with the top-notch education they receive at UCSB, will make students more attractive to biotechnology and bio-pharma recruiters.
The funding is also being used to hire staff — PhD-level project scientists — to manage the user facilities and train students. Equipment such as mass spectrometers, centrifuges, and reagents has also been purchased to complement the robotic assembly systems. O’Malley expects the equipment to be ready to use in the second half of 2024, and hopes that it will bring together researchers from across campus, including those in the fields of biological engineering, earth science, chemistry, ecology, and marine biology, and serve as another beacon for innovative research spawned from the university’s tradition of collaboration and shared facilities.
“This instrumentation will make a transformational impact and unite everyone on campus who works on cells. It enables researchers to think in a new dimension, to design and execute large-scale experiments that push our limits of scientific understanding and develop technology in ways that were not possible before,” said O’Malley, who adds that UCSB will continue to explore additional funding to sustain and enhance the BioFoundry. “The life sciences have not had infrastructure that unites everybody on this scale, similar to how the Materials Research Laboratory exists for the materials community. This also provides a big feather in the cap of the new Bioengineering Department.”